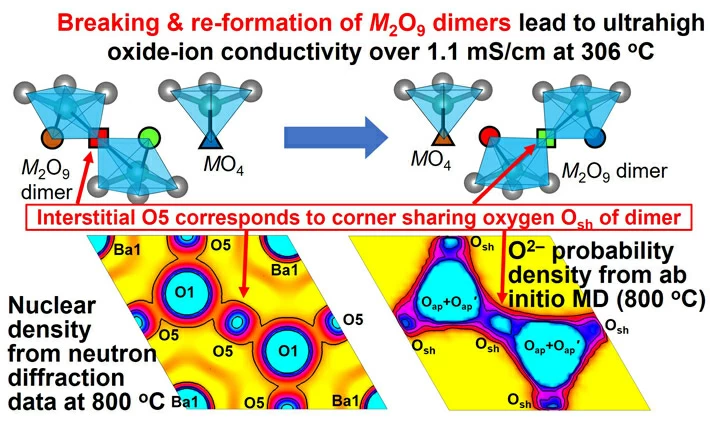
The top figure shows the snapshot for the oxide-ion migration. The red and green oxide ions move by breaking and reforming of M2O9 dimers, which enables fast oxide-ion diffusion where the M cation is Nb5+ or Mo6+. The neutron scattering length density distribution from neutron diffraction data at 800°C in the bottom left figure agrees with the time- and space-averaged probability density distribution of oxide ions from ab initio molecular dynamics simulations in the bottom right figure. The interstitial O5 atom in the bottom left figure corresponds to the corner-sharing oxygen atom (Osh in the bottom right figure and squares in the top figure).
The remarkable proton and oxide-ion (dual-ion) conductivities of hexagonal perovskite-related oxide Ba7Nb3.8Mo1.2O20.1 are promising for next-generation electrochemical devices, as reported by scientists at Tokyo Tech. The unique ion-transport mechanisms they unveiled will hopefully pave the way for better dual-ion conductors, which could play an essential role in tomorrow’s clean energy technologies.
Clean energy technologies are the cornerstone of sustainable societies, and solid-oxide fuel cells (SOFCs) and proton ceramic fuel cells (PCFCs) are among the most promising types of electrochemical devices for green power generation. These devices, however, still face challenges that hinder their development and adoption.
Ideally, SOFCs should be operated at low temperatures to prevent unwanted chemical reactions from degrading their constituent materials. Unfortunately, most known oxide-ion conductors, a key component of SOFCs, only exhibit decent ionic conductivity at elevated temperatures. As for PCFCs, not only are they chemically unstable under carbon dioxide atmospheres, but they also require energy-intensive, high-temperature processing steps during manufacture.
Fortunately, there is a type of material that can solve these problems by combining the benefits of both SOFCs and PCFCs: dual-ion conductors. By supporting the diffusion of both protons and oxide ions, dual-ion conductors can realize high total conductivity at lower temperatures and improve the performance of electrochemical devices. Although some perovskite-related dual-ion conducting materials such as Ba7Nb4MoO20 have been reported, their conductivities are not high enough for practical applications, and their underlying conducting mechanisms are not well understood.
Against this backdrop, a research team led by Professor Masatomo Yashima from Tokyo Institute of Technology, Japan, decided to investigate the conductivity of materials similar to Ba7Nb4MoO20 but with a higher Mo fraction (that is, Ba7Nb4-xMo1+xO20+x/2). Their latest study, which was conducted in collaboration with the Australian Nuclear Science and Technology Organisation (ANSTO), the High Energy Accelerator Research Organization (KEK), and Tohoku University, was published in Chemistry of Materials.
After screening various Ba7Nb4-xMo1+xO20+x/2 compositions, the team found that Ba7Nb3.8Mo1.2O20.1 had remarkable proton and oxide-ion conductivities. “Ba7Nb3.8Mo1.2O20.1 exhibited bulk conductivities of 11 mS/cm at 537°C under wet air and 10 mS/cm at 593°C under dry air. Total direct current conductivity at 400°C in wet air of Ba7Nb3.8Mo1.2O20.1 was 13 times higher than that of Ba7Nb4MoO20, and the bulk conductivity in dry air at 306°C is 175 times higher than that of the conventional yttria-stabilized zirconia (YSZ),” highlights Prof. Yashima.
Next, the researchers sought to shed light on the underlying mechanisms behind these high conductivity values. To this end, they conducted ab initio molecular dynamics (AIMD) simulations, neutron diffraction experiments, and neutron scattering length density analyses. The neutron diffraction experiments at KEK/J-PARC were carried out by Masato Hagihala’s group with SPICA and SuperHRPD diffractometers. These techniques enabled them to study the structure of Ba7Nb3.8Mo1.2O20.1 in greater detail and determine what makes it special as a dual-ion conductor.
Interestingly, the team found that the high oxide-ion conductivity of Ba7Nb3.8Mo1.2O20.1 originates from a unique phenomenon (Figure). It turns out that adjacent MO5 monomers in Ba7Nb3.8Mo1.2O20.1 can form M2O9 dimers by sharing an oxygen atom on one of their corners (M = Nb/Mo cation). The breaking and reforming of these dimers gives rise to ultrafast oxide-ion movement in a manner analogous to a long line of people relaying buckets of water (oxide ions) from one person to the next. Furthermore, the AIMD simulations revealed that the observed high proton conduction was due to efficient proton migration in the hexagonal close-packed BaO3 layers in the material.
Taken together, the results of this study highlight the potential of perovskite-related dual-ion conductors and could serve as guidelines for the rational design of these materials. “The present findings of high conductivities and unique ion migration mechanisms in Ba7Nb3.8Mo1.2O20.1 will help the development of science and engineering of oxide-ion, proton, and dual-ion conductors,” concludes a hopeful Prof. Yashima.
We hope further research leads us to even better conducting materials for next-generation energy technologies.
REFERENCE
Authors | Yuichi Sakuda1, Taito Murakami1, Maxim Avdeev1,2,3, Kotaro Fujii1, Yuta Yasui1, James R. Hester2, Masato Hagihala4, Yoichi Ikeda5, Yusuke Nambu5,6,7, and Masatomo Yashima1 |
Title of original paper: | Dimer-Mediated Cooperative Mechanism of Ultrafast-Ion Conduction in Hexagonal Perovskite-Related Oxides |
Journal: | Chemistry of Materials |
DOI: | 10.1021/acs.chemmater.3c02378 |
Affiliations: | 1Department of Chemistry, School of Science, Tokyo Institute of Technology 2Australian Centre for Neutron Scattering, Australian Nuclear Science and Technology Organisation (ANSTO) 3School of Chemistry, The University of Sydney 4Institute of Materials Structure Science, High Energy Accelerator Research Organization (KEK) 5Institute for Materials Research, Tohoku University 6Organization for Advanced Studies, Tohoku University 7FOREST, Japan Science and Technology Agency |
About Tokyo Institute of Technology
Tokyo Tech stands at the forefront of research and higher education as the leading university for science and technology in Japan. Tokyo Tech researchers excel in fields ranging from materials science to biology, computer science, and physics. Founded in 1881, Tokyo Tech hosts over 10,000 undergraduate and graduate students per year, who develop into scientific leaders and some of the most sought-after engineers in industry. Embodying the Japanese philosophy of “monotsukuri,” meaning “technical ingenuity and innovation,” the Tokyo Tech community strives to contribute to society through high-impact research.
https://www.titech.ac.jp/english/
About High Energy Accelerator Research Organization (KEK)
KEK was established to promote various types of researches as a center of excellence for overall development of Japan’s accelerator science (particle and nuclear research using high energy accelerators, research on the structure/function of materials including living organisms, research on improving the accelerator performance, and related basic technologies). As the Inter-University Research Institute Corporation, KEK provides researchers across the country and abroad with opportunities for research. With the Tsukuba and Tokai campuses as centers for excellence, KEK joins international collaboration experiments and developments. In addition, KEK is in charge of the School of High Energy Accelerator Science of the Graduate University for Advanced Studies (SOKENDAI).
https://www.kek.jp/en/
About Japan Proton Accelerator Research Complex (J-PARC)
J-PARC is a high intensity proton accelerator facility, and it aims at the frontier in materials and life sciences, nuclear and particle physics. It is a joint project between KEK and Japan Atomic Energy Agency. SPICA and SuperHRPD are installed at the Materials and Life Science Experimental Facility (MLF) of J-PARC.
https://j-parc.jp/c/en/index.html
Please refer to the press release for details.